4 How space activity benefits society and contributes to key policy areas
The number of sectors touched in some way by space activities has been increasing in the past few decades. Norwegian authorities use satellites to address key public responsibilities related to sustainability, security and national sovereignty. Satellite-based infrastructure has become a significant factor in value creation across much of the economy, not least by facilitating safe, efficient operations in fields like transportation and natural resource extraction.
Norwegian space policy has been based in large part on finding concrete solutions to society’s needs. Because of the country’s geography and business interests, it became apparent long ago that Norway, more than many other countries, faced national challenges best addressed through space-based technology. Norwegian authorities, researchers and technology companies have often been quick to identify the opportunities represented by new space-related technologies. Today’s advanced satellite systems for ship communications, ocean monitoring and high-precision navigation demonstrate how early-phase Norwegian involvement in promising new technology projects can result in beneficial services and commercial success.
More and more tasks these days can be accomplished cost-effectively by using space-based infrastructure. Moreover, many of the challenges that space-based technology may be helpful in solving have grown in significance in recent years. While Chapter 3 dealt with the political priorities that have shaped Norway’s current approach to space, this chapter will look ahead to the role space activities may play in solving the country’s present and future challenges. In some detail, it examines five policy areas where the use of space-based systems may be particularly cost effective: the High North, climate and environment, security, transport and research.
4.1 The High North
For several decades, the High North has been a central focus of Norwegian space policy. The Government’s space efforts are also discussed in Report No. 22 (2008–2009) to the Storting: Svalbard and Meld. St. 7 (2011–2012) The High North: Visions and strategies. Our needs in the High North have been a key reason for Norway’s commitment to the advancement of ocean monitoring technology through ESA, Copernicus and our radar collaboration with Canada. One of the most important goals of Norwegian participation in the EU satellite navigation programmes EGNOS and Galileo has been to make sure the programmes are able to perform satisfactorily in the High North. The satellite ground operations at Jan Mayen Island and Svalbard are representative of Norway’s continuing involvement in the Arctic, and they help secure the deployment of satellite systems that perform capably over Norway’s ocean expanses.
There are few if any alternatives to satellite-based systems when addressing the communication, navigation, surveillance and preparedness needs of the High North. Distances are too vast and human activities too scattered for land-based infrastructure, aircraft and ships to be effective. Satellite-based infrastructure provides coverage across wider areas. Once such infrastructure is rolled out, the cost of adding users to a service is very low. Radar satellites and AIS satellites can perform their observations day and night regardless of visibility conditions – an obvious advantage in harsh environments far to the north. Still, the High North poses special space-based infrastructure challenges. For some kinds of infrastructure, being on top of the world is an advantage. Satellites in polar orbit provide particularly good coverage near the poles. That’s why ocean monitoring of the High North is one of their strong suits. For other types of infrastructure, northern geography is a challenge. Satellites that move in geostationary orbit, as most communications satellites do, have limited coverage north of 75 degrees latitude. Communicating by satellite in the High North therefore poses special demands to the infrastructure employed.
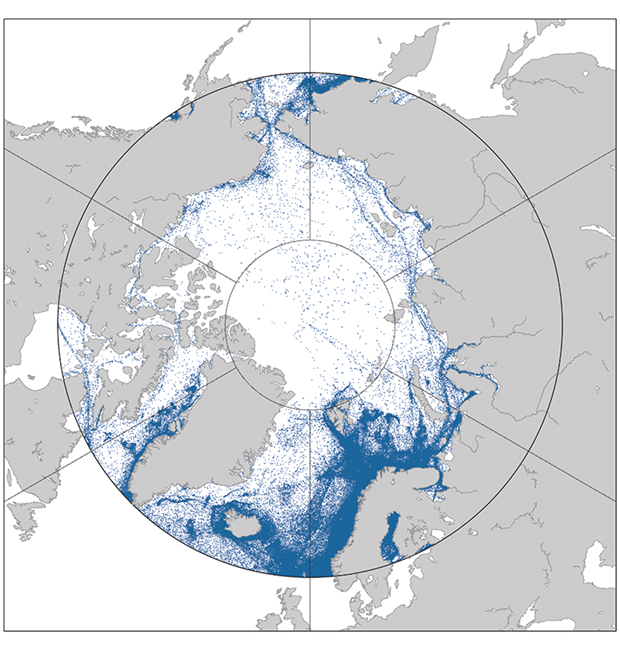
Figure 4.1 Ship traffic north of 60 degrees latitude (1 Oct. 2010–1 Oct. 2012)
Source Norwegian Space Centre/Norwegian Coastal Administration
Activity in the High North and the Arctic is on the rise. Norway’s fishing fleet has been ranging farther and farther northward, and boats off Svalbard have crossed 80 degrees latitude on several occasions. Cruise tourism around Svalbard has grown in scope over the years. The offshore oil and gas industry is moving ever further into the Arctic, while the retraction of Arctic sea ice is increasing the feasibility of long-distance central Arctic Ocean shipping routes. Safe, efficient and sustainable shipping and natural resource extraction in the High North and Arctic will depend in large part on the use of satellite-based infrastructure. The great distances to be traversed and the lack of land-based infrastructure make satellite-based surveillance, navigation and communication essential to safe, efficient ship traffic and sea rescue operations. Advanced offshore petroleum exploration and drilling projects depend on navigation and broadband services available only by satellite. Norwegian authorities already have substantial experience using satellites to monitor oil spills and oversee fisheries. Now, High North shipping is on the rise, the operational radius of the fishing fleet is expanding, and oil and gas companies are entering sensitive environments as the authorities open new zones in and around the Arctic to petroleum activity. The need for improved monitoring is thus likely to intensify, underlining the importance of having high-quality communications and surveillance systems in place.
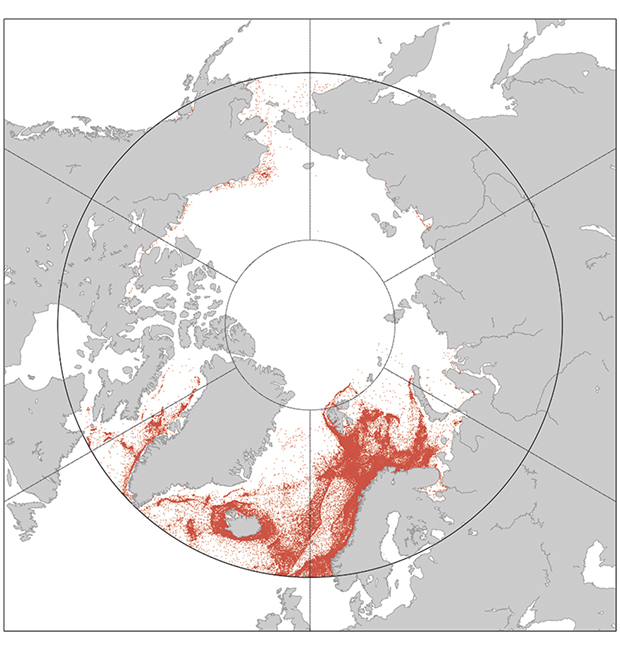
Figure 4.2 Fishing north of 60 degrees latitude (1 Oct. 2010–1 Oct. 2012)
Source Norwegian Space Centre/Norwegian Coastal Administration
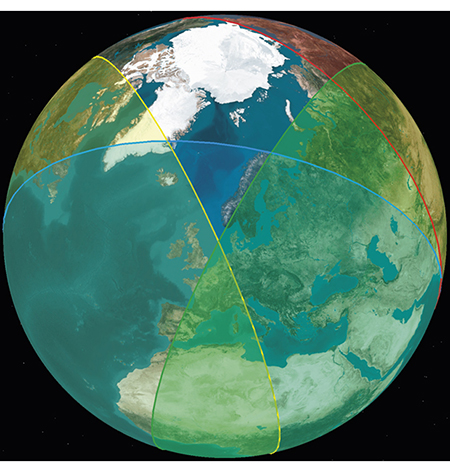
Figure 4.3 Fishing north of 60 degrees latitude (1 Oct. 2010–1 Oct. 2012)
Source Norwegian Space Centre/Norwegian Coastal Administration
The EU satellite navigation programmes Galileo and EGNOS, in combination with the American GPS system and Russia’s GLONASS, will provide robust satellite navigation coverage on land and at sea in the High North and Arctic. Norway’s participation in Galileo’s early phase has helped ensure that the system will perform better in the High North than had originally been planned. As to earth observation capacity in the region, the EU’s Copernicus programme in combination with Norway’s AIS and Canada’s radar satellites should do an adequate job in the years ahead. Good communication systems for the High North, however, remain unprovided for; lacking in particular are broadband services for ships. Existing satellite communication systems offer little or no coverage north of 75 degrees latitude. Communication satellites orbiting over the poles can solve this challenge. Several states with High North interests have started to study options for polar satellite communications, but for now, no plans are ready to implement. In Norway, the Norwegian Space Centre and Telenor have begun the Arctic Satellite Communications project (Norwegian acronym: ASK). The project will examine potential commercial demand and Norwegian governmental needs as well as the relevant communications technologies, organisational structures, costs and funding needs. Such a development may generate activity in northern Norway while reinforcing the Government’s High North strategy.
Textbox 4.1 Satellites
Satellites are the foundation of all applied services that take advantage of space. More than 6,500 satellites have been launched since 1957. About 2,500 satellites currently orbit the earth, and between 800 and 1,000 of them are in active use. There are three main types of satellites, categorised by function. Communication satellites relay signals for TV, telephone and broadband service. Navigation satellites determine position, speed and precise time. Earth observation satellites register data on sea, land and air conditions. The satellites make use of different types of orbits:
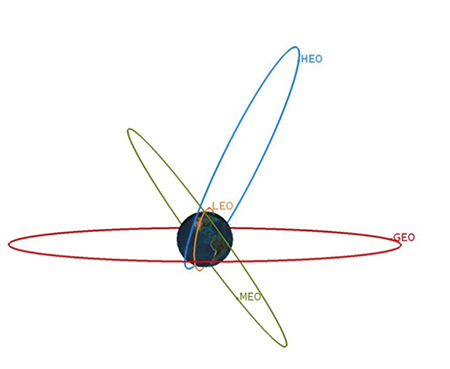
Figure 4.4 Illustration of different satellite orbits
Source Norwegian Space Centre
Satellites in geostationary earth orbit (GEO) move at the same velocity as the earth’s rotation, some 36,000 km over the equator. Their coverage area on earth ranges in latitude from 75 degrees south to 75 degrees north. The polar areas are left uncovered. From the earth’s surface, such satellites appear to be stationary. They are used for TV broadcasting, telecommunications and earth observation.
Navigation satellites occupy medium earth orbit (MEO). These satellites move some 20,000 km above the earth’s surface. Their orbit is inclined at an angle of about 60 degrees from the equator. Circling the earth takes 12 to 14 hours. A minimum fleet of 24 satellites is needed to ensure that four or more satellites have line-of-sight to a given surface location, so that geographical position can be calculated.
Satellites moving in low earth orbit (LEO) have almost circular paths at altitudes of 200 km to 1,500 km. Due to this low altitude, each satellite covers only a small area, whose boundaries shift continuously with the motion of the satellite. Each orbit takes 90 to 120 minutes, and the satellite stays within a user’s line of sight for less than 20 minutes. Many satellites are required to achieve continuous coverage over a fixed area. LEO satellites are used for research, earth observation and communications.
A low-altitude satellite in polar orbit passes over or near the poles. Because of the earth’s rotation, such a satellite can cover the entire surface of the earth in the course of relatively few orbits. At northern latitudes, each orbital passage can be observed.
Satellites in highly elliptical orbit (HEO) move around the earth at varying altitudes. These satellites are used for communications during the long orbital segment when they are furthest from earth. Those that spend most of their time over northern latitudes provide good coverage of the High North. Such orbits typically take 12, 16 or 24 hours.
BarentsWatch is a web portal that collects information on coastal and marine areas from a variety of Norwegian governmental data sources, and makes it available. Though headed by the Norwegian Coastal Administration (NCA), BarentsWatch is beholden to no one agency. It is being developed in phases. The first version of the open information portal was launched in 2012. As services in the open system are gradually rolled out, a closed system for operational purposes is also to be developed. The closed system will combine information from various sources to improve the situational awareness of public maritime authorities. Over time, BarentsWatch is to become a comprehensive monitoring and information system for maritime and coastal areas.
The open portal, Barentswatch.no, will assemble facts and documentation that exist already, but it will also develop new web services that exploit combinations of data from numerous sources. The web portal will offer factual information of a historic nature – statistical data stored systematically over time – but also (near) real-time data reflecting current snapshots of certain activities, phenomena or geographic locations. Users will thus be able to follow developments over time as well as to observe what’s happening at the moment.
The portal will assemble and present material relevant to five major subject areas:
Maritime transport
Marine resources
Oil and gas
Exercise of public authority/sovereignty
Climate/environment
For target groups with interests in one or more of these areas, the portal will be a useful new tool. Information that previously was spread across many sites will be available in one place, and can be combined to achieve a new level of awareness.
4.2 Climate and environment
For years, climate and environmental issues have been rising on the political agenda, both in Norway and internationally. With greater political interest follows the need for increased knowledge to ensure that climate and environmental policy decisions can be made on the basis of sound information. Earth observation satellites have proved to be a particularly suitable tool for obtaining information crucial to our understanding of climate changes and other environmental issues.
The great advantage of using satellites to study environmental and climate-related issues is that they permit observation of large areas as well as repetitive scans of the same spots over time. The earth’s climate is regulated by a variety of large, dynamic phenomena, including solar activity, greenhouse gas emissions, changes in snow and ice cover, atmospheric soot particles, ocean currents, wind systems and variations in cloud cover, to name but a few. Even a partial understanding of the causes and effects of climate changes requires a sense of the complex interplay among these and other phenomena over time. Comparable observations from around the globe, collected over a long period, are therefore necessary.
Textbox 4.2 The Government’s Climate and Forest Initiative
During the climate negotiations in Bali in 2007, the Government decided to allocate up to NOK 3 billion annually for measures designed to reduce greenhouse gases in the atmosphere by preventing deforestation and forest degradation in developing countries. The Climate and Forest Initiative was established in 2008 and is administered today by the Ministry of Foreign Affairs and the Ministry of Environment. Verifying that recipient countries implement what they agree to do is a significant challenge. Satellites are a useful way to document deforestation and forest degradation. From 2009 to 2012, the Norwegian Space Centre led and coordinated Norway’s involvement in the Group on Earth Observations’ Forest Carbon Tracking Task (GEO FCT) and the Global Forest Observations Initiative (GFOI). GFOI is a platform to foster sustained access to satellite- and ground-based observations as well as to support the use of such observations in national forest information systems. The aim is to provide systematic access to satellite data for countries with tropical forests, enabling them to improve their surveillance and anti-deforestation programmes.
In the past 25 years, we have seen a rapid expansion of satellite observation capability. It is now possible to observe most of the key factors influencing the climate. A number of indicators and illustrations presented by the U.N.’s Intergovernmental Panel on Climate Change (IPCC) are based on satellite data, much of it received in Svalbard. The overview provided by satellite observation is critical to monitoring environmental problems such as desertification, oil spills and the long-range transport of pollution. Where terrestrial infrastructure is poor, as in the polar regions and in developing countries, satellite observations are particularly important. They are also used to verify that individual countries fulfil their binding international agreements.
Textbox 4.3 Oil spills, viewed from space
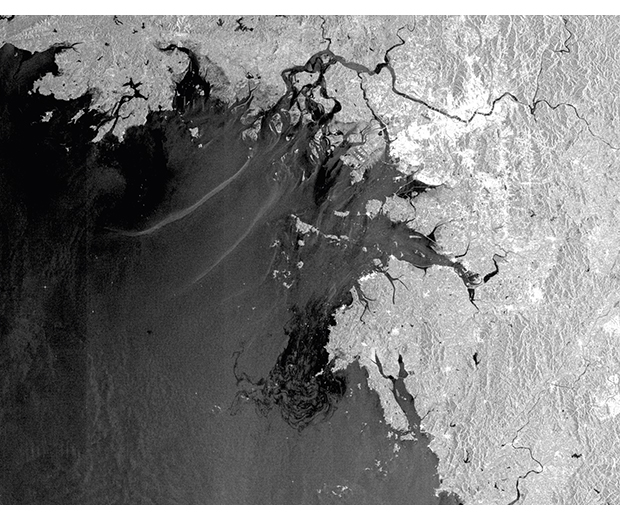
Figure 4.5 Satellite picture of oil spill off South Korea
Source ESA
Radar satellites can «see through» clouds and at night, and they cover large areas. Norway in the 1990s was the first country in the world to employ such satellites on a regular basis to monitor oil spills at sea. At KSAT, in Tromsø, satellite images from many parts of the world are analysed in a search for oil spills from ships or platforms. This form of observation has been developed jointly by Norway’s authorities, research organisations and industrial companies.
4.3 Civil protection, preparedness and crisis response
Satellite-based infrastructure is employed in a wide range of crucial tasks. Communications, navigation and surveillance via satellite have become very important to the performance of safety- and emergency-related public services ranging from sea rescues to oil-spill preparedness and crisis management. Satellite usage gives rise to new and cost-effective means of handling many of our society’s vulnerabilities. At the same time, our satellite dependence carries with it a new type of risk. Saboteurs and cyber attackers, for example, or natural phenomena such as space weather, could cause significant harm by knocking out critical satellite infrastructure. Increasingly, Norway and major international space powers such as the EU and the United States acknowledge the importance of managing this risk.
The utility of satellite-based infrastructure in civil protection, emergency preparedness and crisis management stems from many of the same factors that make satellites useful in addressing needs related to the High North and climate and environmental policy. Satellite communications provide coverage in areas where no other communications systems reach. The value of satellite communications is also demonstrated when storms or other natural disasters damage terrestrial communications infrastructure. In the aftermath of the big Nordic storm «Dagmar», in December 2011, satellite communication systems were the only way to contact the outside world for many people in Norway’s Sogn og Fjordane County. Earth observation satellites provide a quick view of disaster areas and make it easier to determine the appropriate response. Japanese authorities made extensive use of satellite observations to coordinate relief efforts after the Fukushima accident in March 2011. Radar satellites are becoming very important to the mapping and monitoring of unstable mountainsides in Norway.
Textbox 4.4 Satellite-based landslide mapping and monitoring
New satellite sensors make it possible to detect movements of the earth’s surface amounting to just millimetres per year. This has made it possible to develop effective techniques for mapping and monitoring of unstable geological formations, and potentially of road surfaces and railway tracks susceptible to ground movement. Satellite data will increase our knowledge of landslide dynamics and triggering mechanisms, so that advance notice of such events will become more common.
As with all systems our society becomes dependent on, the increased reliance on satellite-based systems represents a potential vulnerability. As satellite systems become more and more important to aerospace, emergency preparedness and security-related activities, it is to be expected that those systems will become attractive targets for hostile attack. Satellite infrastructure can also be damaged by natural phenomena, including space weather, and by space debris. Measures to address such vulnerabilities have become an important governmental priority. The Norwegian Space Centre is in the process of preparing a vulnerability report on the civilian use of satellite navigation systems. The report was ordered by the Ministry of Fisheries and Coastal Affairs, which is responsible for coordinating Norway’s civilian radio-navigation policy. The development of potential alternatives to the GPS satellite navigation system – Galileo, GLONASS and Beidou – will help reduce the vulnerability that comes with dependence on a single system. The «Public Regulated Service» component of the EU’s Galileo programme is designed to provide national emergency service providers with access to encrypted satellite navigation services. These will be less vulnerable to attempts at sabotage or manipulation. With regard to space debris, the EU and the United States are pushing to establish international guidelines aimed at reducing the amount of space debris in key satellite orbits.
Textbox 4.5 Space debris
The number of objects in earth orbit has increased significantly over the past decade due to collisions and explosions. The orbits of nearly 20,000 objects are followed regularly by radar or telescope. Many additional orbiting objects are large enough to damage satellites, but too small to be tracked from the ground. The orbital speed is typically 7 km per second. With increasing frequency, operative satellites must be manoeuvred in their orbits to avoid collision with space debris. That requires fuel and reduces the lifespan of an orbiting satellite. The United States is the leading operator of space surveillance systems, and regularly transmits collision alerts to satellite owners in many other countries. A European capacity to contribute to space surveillance is under construction. The United Nations has also become involved in this field. Radar systems in northern Norway and Svalbard already contribute to efforts to obtain a clearer view of the traffic in space. It is hoped that larger satellites will be steered out of orbit when their operative periods end. Such end-of-life operations are not always successful. ESA’s large environmental monitoring satellite ENVISAT suddenly stopped working in the spring of 2012, and will continue orbiting for more than 100 years if not removed by some other means.
4.4 Safe, efficient transport
Transport is one of the sectors that has changed the most in recent years as a result of the increased use of satellite-based infrastructure. It is also the sector whose use of satellite systems is most evident in daily life. Car navigation systems and handheld navigation devices are examples that come quickly to mind, but the transport sector relies on satellite-based infrastructure for communication and earth observation services in addition to navigation. According to the white paper Meld. St. 26 (2012–2013) National Transport Plan 2014–2023, the Government’s overall objective for transport policy is to provide an efficient, accessible, safe and environmentally friendly transport system that meets the transport needs of society and promotes regional development. Satellite-based services are important to maritime, aviation and land-based transport activities, and are classified as intelligent transport systems and services (ITS).
Textbox 4.6 ITS (intelligent transport systems and services)
The acronym ITS (intelligent transport systems and services) has evolved into a generic term for a wide range of technological approaches to improving transportation, in particular those focused on information and communications technology. Examples include systems for road-traffic information dissemination, satellite navigation, electronic ticketing and logistics. ITS can help increase capacity utilisation and efficiency across the entire transport sector: roads, rail, sea, and aviation.
Source Ministry of Transport and Communications, Strategy for intelligent transport systems, 2010)
Maritime transport
In maritime transport, satellites are crucial to ship owners who require contact with their ships. Satellites also let crew members to talk to their families, watch television and access the Internet. Indeed, for ship crews far from land, satellite links are the only way to communicate with the outside world. To improve safety at sea, carriage requirements mandate the installation of satellite-based communications and navigation systems on larger vessels. Satellite data are also important for the design of maritime navigational charts. Satellite data are also used to map the current status of ice cover, so that ship crews in northern waters can plot cost-effective routes. Norway, an active participant in the UN International Maritime Organisation (IMO), built a chain of AIS (Automatic Identification System) receivers along the Norwegian coast soon after the IMO enacted its resolution introducing AIS. Such coastal networks in concert with satellite-based AIS technology provide a clear overview of global traffic at sea. Data from the Norwegian AIS satellite and the Norwegian AIS receiver on the International Space Station have made valuable contributions to the fight against piracy in the waters off Africa. Satellites also allow Norwegian authorities to substantially improve their supervision of vessel activity in the South Atlantic, including the waters around Bouvet Island and off Dronning Maud Land.
Aviation
Many Norwegian aircraft today use GPS for horizontal navigation during parts of their flights. GPS is the primary source of navigational data for the helicopter traffic that carries 600,000 passengers a year to and from North Sea installations. While flying in this airspace, helicopters broadcast their GPS-derived positions to ground stations. The use of this technology for monitoring and controlling air traffic halves the cost in comparison with construction of radar systems. The Norwegian air ambulance helicopter service uses GPS-based procedures on approach to bases and hospitals. Satellite-based landing systems allow aircraft crews to land and take off in curving trajectories, to plan the most direct flight paths, to save energy, and to modify approach paths so as to minimise the noise burden for airport neighbours. Runway-length needs can also be reduced – a benefit for airports in northern Norway in particular. Of late, GPS has been used increasingly to provide vertical navigational assistance, especially during airport approach and departure and other situations where the system provides operational and environmental benefits. Satellite technology is an element of initiatives like Performance Based Navigation, which is being phased in here in Norway and elsewhere.
Satellite-based infrastructure assists aviation in many ways. When Iceland’s Eyjafjallajökull volcano erupted in 2010, airborne volcanic ash created major problems for aircraft. Millions of passengers experienced delays, and even air ambulance services were grounded. The eruption inflicted major economic losses on airlines. But the following year, when Iceland’s Grimsvötn volcano erupted, Norwegian authorities were able to hold large swaths of airspace open. By analysing detailed satellite observations and weather models, they were able to minimise travel delays and economic losses.
Textbox 4.7 Volcanic eruption at Eyjafjallajökull
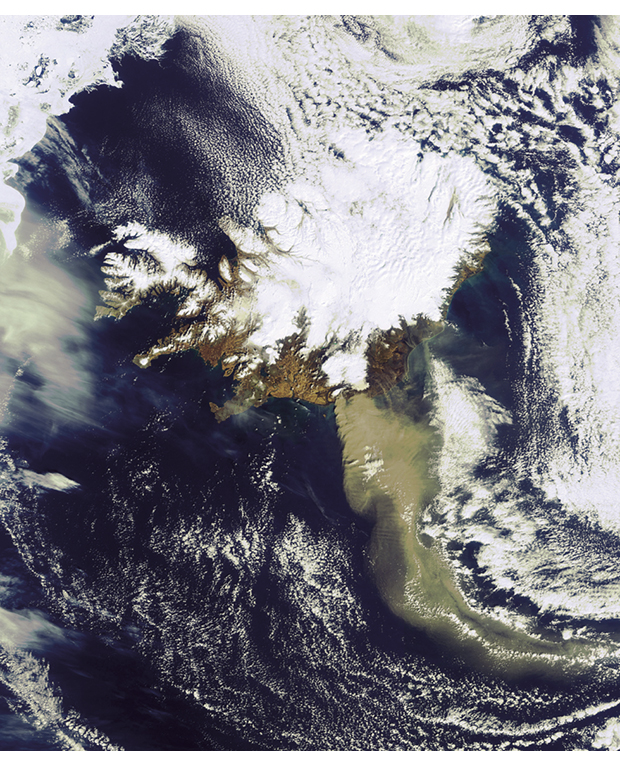
Figure 4.6 Eruption at Eyjafjallajökull
Source ESA
The April 2010 eruption of the Eyjafjallajökull volcano in Iceland had a major impact on air travel across Europe. In May 2011, however, during the eruption of Grimsvötn, Norwegian airspace could be held open. The country was thus spared major economic losses. Satellite imagery and weather models were key to the operational decision-making in 2011.
Land transport
Satellite-based infrastructure for navigation is very important to land transport. In 2010, 32 per cent of vehicles in the EU made use of satellite navigation. The Norwegian Public Roads Administration relies on satellite-based navigation and data services to perform road construction and maintenance. New forms of road pricing that rely on satellite navigation sensors are potentially more accurate than those that use existing ground-based tolling systems. Satellite navigation is also employed in fleet management and package tracking, enabling freight companies to plan their loading and unloading operations in advance and to alert customers when to expect delivery.
For rail systems, GPS data is more valuable than physical detection systems for calculating train speed and station arrival and departure times. Such positioning data is also used in combination with energy data to accurately invoice train operators for their energy consumption on routes crossing national boundaries. GPS devices fitted into locomotives are also used in maintenance planning; they let planners and workshop personnel know at all times where particular equipment is located and how far it has travelled since prior maintenance.
4.5 Research
In a relatively short period, researchers across nearly the whole spectrum of natural sciences have put satellite data to use in important ways. They use satellites to observe everything from wind systems and ice cover to air pollution and forest conditions. Among the great advantages of satellite-based technology are the ability to observe large areas in a cost effective manner and the ability to make many commensurate observations over time. Information obtained from satellites is particularly important to understanding large, dynamic phenomena such as weather systems, long-range migration of pollution and climate changes. Scientific research into the High North is a case in point. In a region characterised by vast distances, poor infrastructure and harsh climate, good alternatives to satellite-based observation are hard to find. Research results from the International Polar Year (2007–2008) show that satellite data are particularly valuable to research in the polar regions.
Norway participates in international space activities through ESA, Galileo and Copernicus, and through a number of bilateral agreements. Such cooperation puts Norwegian researchers in direct contact with their international colleagues and gives them dependable access to data and a say in scheduling future observations. The information obtained and lessons learned help Norway expand its scientific and technological knowledge base in areas of great strategic importance.
Data that can only be obtained from satellites play a major role in the study of processes in, on and around the earth. The oldest field of study is atmospheric dynamics, which is the basis for numerical weather models. Since the early years of the 20th century, when the physicist Vilhelm Bjerknes did pioneering work in meteorology, Norwegian scientists and organisations have been leaders in the development of weather models. More recently they have been in the forefront of climate modelling. Atmospheric models have been expanded to include a wide range of chemical components and reactions, and satellite instruments today measure a range of chemical components in the atmosphere. Observations and research conducted at Ny-Ålesund, in Svalbard, are among Norway’s contributions to international conventions on monitoring global air pollution.
Ocean research is a field where satellite data is crucially important, due to the vast geographic extent of marine areas and the problems of physical access. Leading Norwegian academic and research groups participate in all the major European marine research programmes. Newer satellites, like ESA’s GOCE research satellite, make it possible to include coastal areas in ocean modelling. That’s a development of interest to Norway. Norway is also a leader in sea-ice research and oil-spill detection. The observation of algal blooms to help understand how they develop is also of great importance, not least for the aquaculture industry.
Textbox 4.8 What can satellites tell us about climate and the environment?
At sea: Satellite observation is especially useful over open water, where geometric resolution need not be particularly high. Satellites can measure sea level, sea ice, objects on the sea surface, wave height, currents, ocean temperature, ocean «colour» (associated with algal blooms, for example), particle content (suspended matter), distribution of spilled oil, etc. Wind speed and wind direction at sea level are measured for weather forecasting purposes.
Fresh water – rivers, lakes and groundwater: Satellite observation technology is increasingly applicable to coastlines, fjords and freshwater bodies. The parameters that are measurable at sea are also measureable in freshwater bodies. Limitations apply, however, particularly for small water surfaces.
Land, land use and biodiversity on land: Satellite observation applications ashore are wide ranging, from environmental surveillance of large areas to the details of properties or infrastructure. Satellites equipped with optical and infrared sensors can provide important data on vegetation, including climate-related changes, vegetation cover, land use, forest condition/forest damage/deforestation, pollen, and urban green areas. Radar observation can provide data on landslides, glacier movement, snow, ice and flooding.
Air pollution, both local and global: Satellite observations are used increasingly in combination with in-situ measurements. Techniques to measure concentrations of NO2 , SO2, CO, CH2O and aerosols are well developed. Satellites still lack the accuracy and sensitivity of ground instruments, but their broad spatial coverage makes satellites useful. Satellites measure gas and particle concentrations, making it possible to estimate pollution emissions, monitor trends and issue alerts when appropriate. Detection of volcanic ash is an important application.
Climate and greenhouse gas emissions: Not all gases are equally observable from space, because they have different absorption characteristics. Some greenhouse gases are observable by satellite, however, and products are available to measure CO2, CH4 and H2O.
Ozone, UV and solar radiation: Ways to quantify stratospheric ozone, ozone-depleting substances and related UV and solar radiation are well established. Satellite data are particularly useful in monitoring the size of the Antarctic ozone hole and studying the depletion of ozone in our own areas. Ozone data from satellites (TOMS/OMI) are used operationally in Norway to estimate and forecast UV radiation for the general public.
Polar regions: Satellite observation is unsurpassed as a means to acquire data frequently and quickly on what’s happening in the polar regions. In the years ahead, more than 150 instruments fitted onto various satellites will be able to observe Svalbard. Measuring sea ice is the most obvious application, since reliable measurement is practically impossible without satellite data. In addition to ice extent, thickness and volume, observable features include currents (ice transport) and changes in the sea ice’s role as animal habitat and transport medium. ESA’s CryoSat satellite provides sea-level data for the central Arctic Ocean. Looking down on polar land masses, satellites observe glacier coverage and changes in vegetation. The large flow of data will necessitate a substantial effort to calibrate and validate the various observed parameters, using ground-based measurements.
Cultural heritage: Satellites are used to localise and monitor cultural heritage sites, as a supplement to field work.
Research on land areas is multi-faceted, and Norway has made its mark on research into snow and ice and the use of satellite data for forest research. At the Norwegian Forest and Landscape Institute, researchers have used earth observation of agricultural conditions to develop operative monitoring systems for forests and other outlying resources. Agriculture itself is largely dependent on observations made during the course of a short growing season. Because satellites now under planning will provide expanded observational coverage, researchers envisage potential new applications tied to resource mapping, forest degradation and other changes in vegetation. A long tradition of systematic, field-based sampling in agriculture provides a solid basis on which to develop earth observation services.
Satellites can tell us much about active processes inside the earth. In 2013, ESA will launch SWARM, a system of three satellites designed to observe the earth’s electromagnetic fields. It is believed that such measurements from space will make it possible to answer questions about the earth’s crust and interior. Research centres in Norway are strongly attuned to the earth’s crustal magnetic fields. What they learn helps us to understand developments in Nordic geology as well as to chart natural resources.
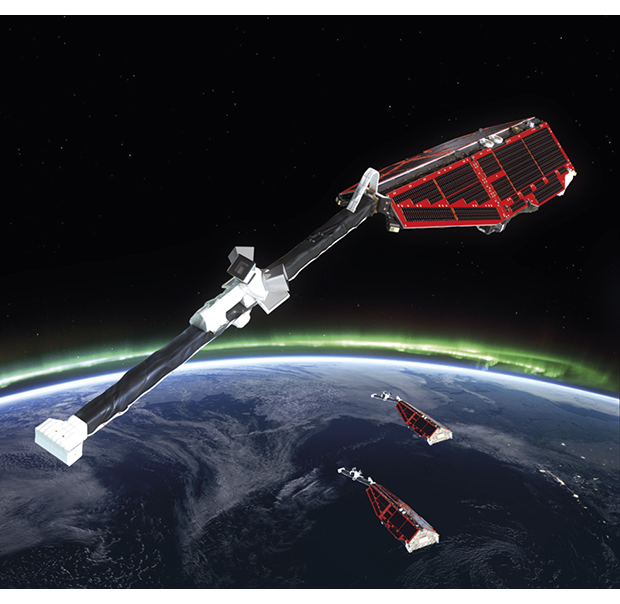
Figure 4.7 SWARM
Source ESA/AOES Medialab
In addition to being a major consumer of satellite data for research, Norway hosts a range of activities associated with the launch of research rockets from launch pads at Andøya and Svalbard. These rocket missions are a necessary complement to satellite and aircraft observations, and are important to understanding phenomena such as the aurora borealis, space weather and climate changes. Norway is one of the few places in the world where rocket-based research can be conducted at Arctic latitudes. International collaboration at Norwegian rocket ranges is extensive.
Norwegian research efforts have provided much of the basis for space-related business growth in Norway. But business development has been characterised by specialisation in clearly defined technical niches. A number of industrial players do operate within the same value chain, though on different levels. This has made collaboration and knowledge sharing possible. Formalised collaboration was attempted through the SIREN space cluster, an ARENA project in the 2006–2009 period. When the project was evaluated, a conclusion was that one and the same value chain lacked critical mass, despite the fact that several strong space-sector participants had been involved in SIREN. The space cluster contributed nonetheless to the founding of the Centre for Remote Sensing in Tromsø, in 2008.
In the field of satellite communications, back in the 1970s and 1980s, Oslo and Akershus counties saw the emergence of a network of companies, many of which had common goals in satellite operations and the development and manufacture of satellite communications equipment. Many of Norway’s technology actors are participants in the Norwegian Centres of Expertise (NCE) programme, which offers collaboration and funding in the speciality disciplines the actors represent. Examples include NCE Instrumentation, NCE Systems Engineering and NCE Micro- and Nanotechnology. In addition, a number of companies and organisations established the Space & Energy Network in 2009 to promote cooperation between the energy and space sectors. The network focuses on open innovation, skills development, technology sharing and high-quality meeting arenas in which to highlight shared technology challenges like extreme operating conditions, remote control, automation, materials selection, project management and safety.